Finite Element Analysis of Friction Stir Welding of Al Alloy and Inconel 718
Friction Stir Welding (FSW) is a solid-state joining technique that has become increasingly popular and efficient for welding both similar and dissimilar metallic materials. Despite its success in joining dissimilar materials, further development is necessary to meet industry requirements and improve understanding of the FSW process. The welding of nickel-based superalloys such as Inconel 718 presents technical challenges, with limited literature suggesting that the process is only achievable at high axial forces and selective process parameters, limiting its industrial applications. To address these challenges, this study proposes 3D finite element models that couple multiphysics, including material flow and heat transfer via conduction, convection, and radiation. A 3D thermo-mechanical model was developed in COMSOL Multiphysics® to simulate the FSW process. The model was validated using experimental results for Al alloys and extended to Inconel 718. The models were used to investigate the temperature and strain distribution in the nugget region, thermo-mechanically affected region, and heat-affected region. The results provide a comprehensive understanding of the FSW process at certain process parameters, including rotational speed, welding speed, and normal force on performance indicators such as temperature distribution, average grain size, and microhardness. The FSW process was modeled by using a moving coordinate system fixed to the tool axis. The tool was divided into a pin and a shoulder. Heat fluxes generated by friction between the rotating tool and contact surfaces were considered, with temperature-dependent mechanical and physical properties of the base materials (Al-T6 and Inconel 718) accurately defined. To capture the plastic behavior of the base metal, the Johnson-Cook constitutive model, which accounts for work hardening, thermal softening, and strain rate hardening, was utilized. The model also estimated the average grain size based on the Zener-Hollomon parameter, which was then used to estimate hardness through the Hall-Petch relationship. Mesh independent studies were conducted to determine the minimum mesh size required for accurate results. The study utilized a mesh of 86,000 elements with an average element quality of 0.78. The highest temperature recorded for the Al plates was 676°C at the welding joint, exhibiting a heat dissipation pattern across the aluminum plates ranging from 200 to 600°C. These results emphasize the complexity of heat transfer during the FSW process and the significance of considering temperature distribution for successful welding outcomes. In contrast, the 3D temperature profile of the Inconel plates indicated a peak temperature of approximately 1300°C, localized exclusively to the welding regions. Further insight was obtained from the 2D cross section, revealing a maximum temperature concentration around the welding regions and a relatively uniform distribution in the x-direction. These findings highlight the more focused temperature distribution of Inconel 718 around the welding regions, implying enhanced heat dissipation across the plates. Consequently, Inconel 718 may possess improved resistance to thermal degradation and an extended lifespan in high-temperature applications. This information can be used to optimize the FSW process and improve the quality of the resulting welds.
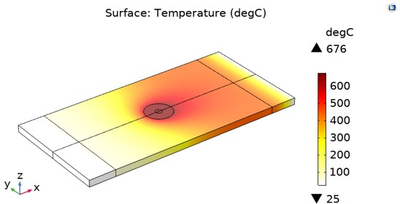